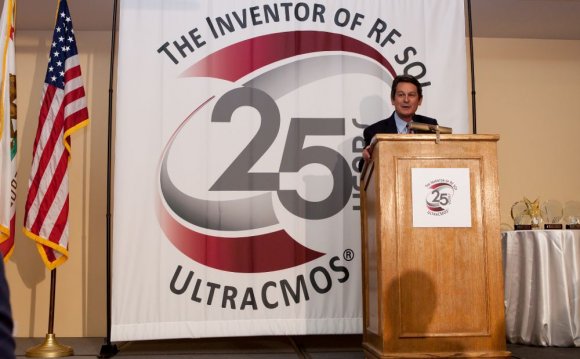
For Detailed Analysis of Semiconductor Devices at the Fundamental Level
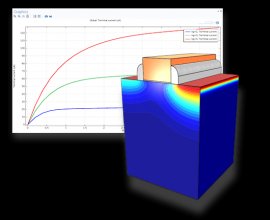
Transistor operation where an applied gate voltage turns the device on and then determines the drain saturation current.
MOSFETs, MESFETs, and Schottky Diodes
The Semiconductor Module allows for detailed analysis of semiconductor device operation at the fundamental physics level. The module is based on the drift-diffusion equations, using isothermal or nonisothermal transport models. It is useful for simulating a range of practical devices – including bipolar, metal semiconductor field-effect transistors (MESFETs), metal-oxide-semiconductor field-effect transistors (MOSFETs), Schottky diodes, thyristors, and P-N junctions.
Multiphysics effects can often have important influences on semiconductor device performance. Semiconductor processing frequently occurs at high temperatures and, consequently, stresses can be introduced into the materials. Furthermore, high-power devices can generate a significant amount of heat. The Semiconductor Module enables semiconductor device-level modeling on the COMSOL platform, allowing you to easily create customized simulations involving multiple physical effects. Moreover, the software is uniquely transparent, as you are always able to manipulate the model equations, leaving you with complete freedom in the definition of phenomena that are not predefined in the module.
Additional images:
- The DC characteristic of a MOS transistor demonstrating transistor operation where an applied gate voltage turns the device on and then determines the drain saturation current.
Make Use of Finite Element or Finite Volume Discretization
You can choose to make use of the finite element or finite volume method when modeling the transport of holes and electrons in the Semiconductor Module. Each method has its set of advantages and disadvantages:
- Finite Volume Discretization: Finite volume discretization in the modeling of semiconductor devices inherently conserves current. As a result, it provides the most accurate result for the current density of the charge carriers. The Semiconductor Module uses a Scharfetter-Gummel upwinding scheme for the charge carrier equations. It produces a solution that is constant within each mesh element, so that fluxes can only be constructed on the mesh faces that are adjacent to two mesh elements. Yet, as products in the COMSOL Product Suite are based on the finite element method, this can make it a bit more challenging to set up multiphysics models.
- Finite Element Discretization: The finite element method is an energy-conserving method. Consequently, current conservation is not implicit in the technique. To obtain accurate currents, it may be necessary to tighten the default solver tolerances or to refine your mesh. In order to help with numerical stability, a Galerkin least squares stabilization method is included when solving the physics in semiconductor devices. One advantage of modeling semiconductor devices with the finite element method is that you can more easily couple your model to other physics, such as heat transfer or solid mechanics, in a single model.
You Can Model All Types of Semiconductors
The Semiconductor Module is used for modeling semiconductor devices with length scales of 100’s of nm or more, which can still be modeled by a conventional drift-diffusion approach using partial differential equations. Within the product, there is a number of physics interfaces – tools for receiving model inputs to describe a set of physical equations and boundary conditions. These include interfaces for modeling the transport of electrons and holes in semiconductor devices, the electrostatic behavior of such, and an interface for coupling semiconductor simulations to a SPICE circuit simulation.
The Semiconductor interface solves Poisson’s equation in conjunction with the continuity equations for the charge carriers. It solves for both the electron and hole concentrations explicitly. You can choose between solving your model with the finite volume method or the finite element method. The Semiconductor interface includes material models for semiconducting and insulating materials, in addition to boundary conditions for ohmic contacts, Schottky contacts, gates, and a wide range of electrostatics boundary conditions.
Features within the Semiconductor interface describe the mobility property as it is limited by the scattering of carriers within the material. The Semiconductor Module includes several predefined mobility models and the option to create custom, user-defined mobility models. Both these types of models can be combined in arbitrary ways. Each mobility model defines an output electron and hole mobility. The output mobility can be used as an input to other mobility models, while equations can be used to combine mobilities, for example using Matthiessen's rule. The Semiconductor interface also contains features to add Auger, Direct, and Shockley-Read Hall recombination to a semiconducting domain, or you can specify your own recombination rate.
Specifying the doping distribution is critical for the modeling of semiconductor devices. The Semiconductor Module provides a Doping model feature to do this. Constant and user-defined doping profiles can be specified, or an approximate Gaussian doping profile can be used. It is also straightforward to import data from external sources into COMSOL Multiphysics®, which can be treated by built-in interpolation functions.
RELATED VIDEO
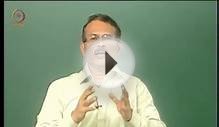
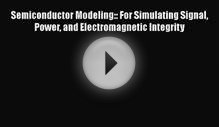
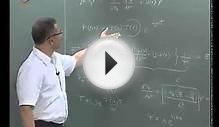